The Proton and its Particles
The interior makeup of protons has been at the center of long debate; new evidence from an experiment known as SeaQuest looks to answer the question of what exactly the proton is made of.
Reading Time: 4 minutes
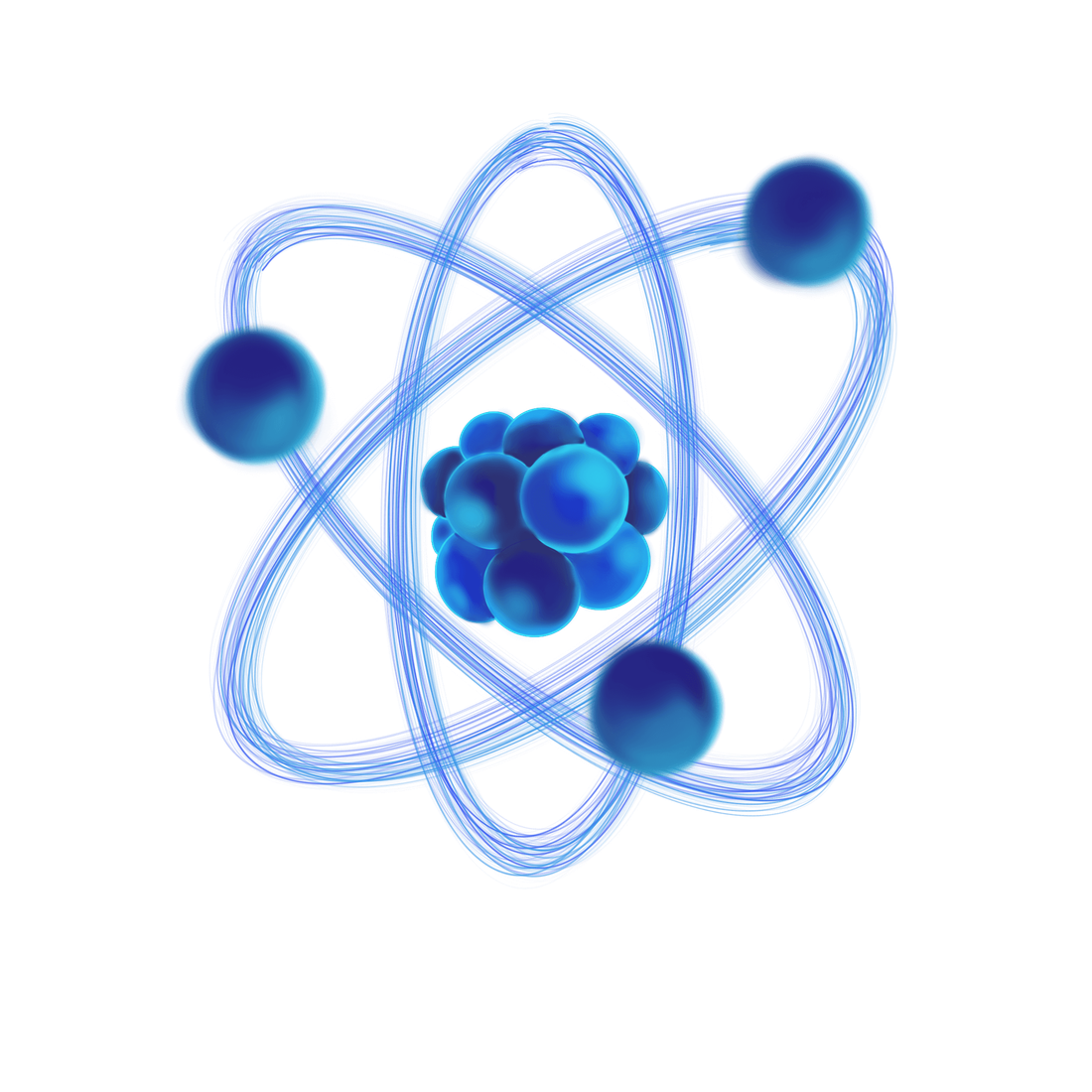
You have probably learned about the proton from your biology and chemistry classes. Residing in the nucleus of the atom, a proton is the positively charged subatomic particle that gives an element its atomic number. As positively charged particles, they are known to attract the negatively charged electrons, leading to atomic interactions.
The cause of the positive charge sounds simple. Protons are composed of smaller subatomic particles known as quarks. These quarks are divided into the subcategories of “up” quarks and “down” quarks with respective electric charges of +2/3 and -1/3. To have a positive charge of +1, scientists believe the proton to have two up quarks (+4/3) and one down quark (-1/3). The quarks are held together by particles called gluons. Gluons use a force, known as the “strong force,” to bind the up and down quarks together and give the proton its characteristic +1 charge.
However, the reasons behind the proton’s positive charge are much more elusive than this. The proton’s interior is also composed of antiquarks, the quark’s antimatter counterpart. Antimatter is the opposite of matter, so antiparticles have magnetic and electrical properties opposite to those of their normal matter particles. For example, quarks have an electrical charge that is opposite to that of the antiquark. The quarks, antiquarks, and gluons collectively form a sea of elementary particles to form the proton.
SeaQuest, an experiment dedicated to exploring and ascertaining the internal makeup of the proton, was completed on February 24, and its results offer more insight into the proton’s interior while uncovering new questions about its makeup. Perhaps the most important result of SeaQuest is that its results support two theoretical models of the proton’s interior. The first is known as the “pion cloud” model. Pions are elementary particles belonging to a group of particles known as mesons, which are composed of one up quark and one down quark. According to the pion cloud model, the proton has a habit of expelling and reabsorbing particles to maintain its internal balance. The second model, known as the “statistical model,” treats the proton like a cloud of gas. However, both indicate a lack of clarity about the proton’s structure. Through experiments like SeaQuest, researchers hope to choose the most appropriate model or even develop newer and more accurate models.
The questions surrounding the proton’s internal sea can be traced back to initial experiments executed to confirm the quark model of subatomic particles. Scientists at Stanford University’s Stanford Linear Accelerator Center (SLAC) confirmed the quark model after they directed high-speed electrons toward protons. The electrons bounced off something inside the proton, suggesting that protons are divisible particles made up of what is now called quarks. However, when SLAC launched higher-speed electrons at the protons, they found that they seemed to bounce off of even more internal particles than originally described: each launch of higher-speed electrons appeared to have produced more internal matter.
Scientists finally began to work towards a more detailed and complex theory about the proton’s internal makeup known as quantum chromodynamics (QCD). QCD describes the strong force of the gluons and how it interacts in the proton's interior. It postulates that gluons split into quark-antiquark pairs. The pairing of matter and antimatter causes these pairs to blink into existence only to blink out into energy as quickly as they cancel each other out.
While the electrons launched by SLAC were hitting the expected quarks, the high-speed electrons also hit the quark-antiquark pairs created by the ocean of gluons. Gluons feel the strong force that they are responsible for, which allows them to proliferate and die out quickly in the form of quarks and their antimatter counterparts. Additionally, scientists inferred that there may be more down antiquarks than up antiquarks, indicating that the proton’s interior is imbalanced through a feature known as asymmetry. Beyond defying original models of the proton, it implies that there is still much unknown about antimatter in the universe.
Thanks to SeaQuest, the mysterious interior of the proton and its asymmetry is shown in a new light, one that better illustrates its nuances and complexities. Since SeaQuest’s final results support the two models of the proton’s interior—the pion cloud model and the statistical model—proponents of either side have been arguing hotly about which model is more accurate. Claude Bourrely, a developer of the statistical model, believes that SeaQuest’s evidence supports his model. In contrast, Mary Alberg and Gerald Miller, who are supporters of the pion cloud model, refer to Bourrely’s model as “descriptive, rather than predictive,” as they believe that it is fine-tuned to the results of SeaQuest’s data. Alberg and Miller both believe that the pion cloud model better accounts for the quark-antiquark pairings while also accounting for other particles’ abilities to bond with one another. From this, they further postulate that the pion cloud model accounts for the ability of nuclei to bond together, explaining greater atomic interactions, perhaps on a molecular level.
To determine the more accurate model of the proton, participants of SeaQuest are on board to launch another experiment called SpinQuest to take into account the spin, or intrinsic angular momentum, of a proton. The term “spin” does not refer to subatomic particles turning rapidly; rather, it describes the way they create small magnetic fields as they orbit around other subatomic particles, which further determines the way they interact with other particles. Spinquest’s specific approach to the proton’s elusive interior is linked to an experiment that indicated that only 30 percent of the proton’s spin is accounted for by the two up-quarks and single down-quark. This means that there are other forces at play that affect the proton’s spin beside the quarks. The SpinQuest experiment hopes to establish what might account for the remaining 70 percent of the proton’s spin. In turn, they hope that the new information about the proton’s spin will allow them to discover a more accurate model for the proton’s interior.
For such a simple subatomic particle, there is much yet to be discovered about how it functions. The next time you hear a mention of protons in your classes, be thankful that you are not tasked with determining what they are made of.