Understanding How Fingerprint Patterns Are Formed
An explanation of the research done to understand how fingerprint patterns are formed through the Turing system.
Reading Time: 4 minutes
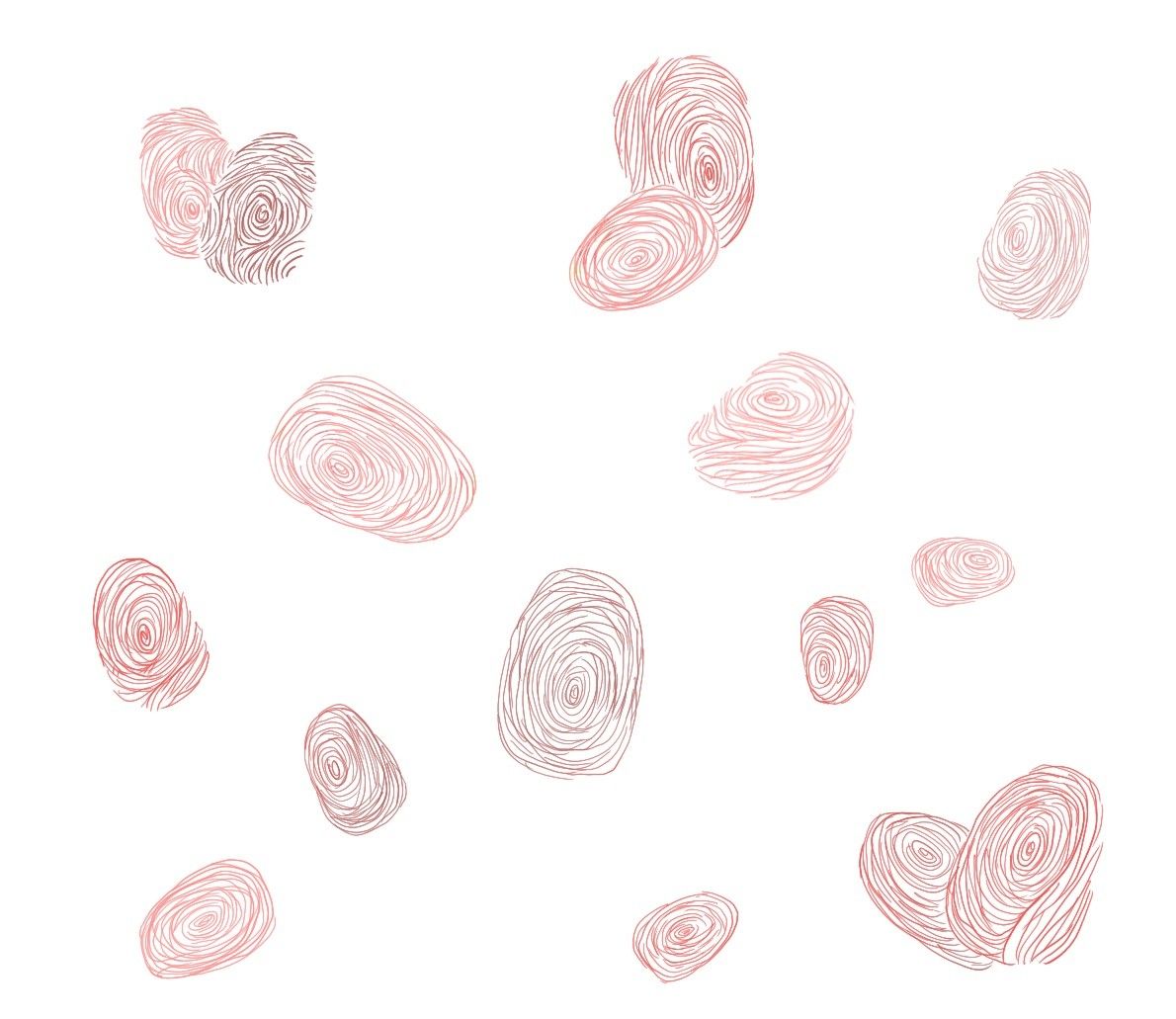
In 1910, Thomas Jennings was brought in as a suspect for the murder of Clarence Hiller, a homeowner killed after a confrontation with an intruder. To prove Jennings was guilty, the police used the fingerprints found on a freshly painted rail, which the intruder utilized to hoist himself into the home. The fingerprints were shown to be identical to Jennings’s, making this case the first criminal trial in American history to convict on the basis of fingerprint evidence.
Fingerprinting is based on friction ridge patterns, or, as Sir Francis Galton coined them, “fingerprint patterns.” Fingerprints are unique from person to person (even identical twins), but all are composed of the same general features: loops recurve unto themselves, whorls are circular patterns, and arches are wavelike patterns. The formation of unique fingerprints is possible through a combination of factors, including genetics and the environment inside the womb during fetal development.
However, the specifics of the fingerprint formation process have always left scientists puzzled; after all, it’s difficult to create an accurate and cost-efficient experiment to determine how fingerprints form because they develop in the early stages of pregnancy. But a team of scientists from the Roslin Institute were able to mimic the development of fingerprints in February 2023 by tracking the genetics behind the developmental formation of transverse digital ridges in fetal mice. Before they began the experiment, the scientists had to understand what molecules they could manipulate in the mice to create different print patterns. Fingerprints form by first growing down into the skin to create valleys—the space between ridges. Then, after a few weeks, the cells at the bottom of these valleys stop growing downward. Instead, the cells in the finger pad begin to push the skin upward to create ridges. To understand what molecules cause this unusual growth pattern, hair follicles were chosen because the team discovered the growth of the two skin structures was nearly identical in its beginning stages.
As expected, the structures shared certain signaling molecules: WNT, EDAR, and BMP. WNT is the molecule that promotes cell growth, leading to the ridges on fingerprints. Cells produce EDAR to boost WNT activity. BMP, on the other hand, restricts and stops these actions, leading to the valleys in fingerprints. As the functions of these three molecules opposed each other to first create downward growth and then upward growth, they formed a Turing reaction-diffusion system—the opposition of an activator and an inhibitor, leading to the formation of unique patterns based on the different diffusion rates.
Next, the researchers tracked the mice’s transverse digital ridge development to experiment with how they led to different fingerprint patterns. The transverse digital ridges in mice toes are striped ridges similar to human fingerprints. Just like fingerprints, these digital ridges also contain Merkel cells—cells below the epidermis that may be involved in the sensation of touch—indicating underlying dermal structures that could be used to form primary ridge patterns. Additionally, the ridges in mice toes are formed at random and express certain molecules like EDAR, which prefigures primary ridge locations just as human fingerprints do. The experiments revealed that EDAR created the distinct ridges, so increasing its presence led to broader, more spaced out ridges, while decreased EDAR led to spots. In contrast, BMP creates valleys, so more BMP led to narrower, closer ridges, and less BMP led to stripes.
Though the researchers believed that human fingerprints followed these rules as well, further evidence was needed to prove it. This tracking only explained some patterns in human fingerprints, as mouse digits are too small to fit the elaborate shapes of human fingerprint patterns. As a solution, the researchers created mathematical models of human fingerprints unto which they could apply Turing’s rules. From the known starting points of fingerprint formation on the finger pad—which are under the nail, the crease of the joint, and the center of the finger pad—the researchers manipulated the timing, locations, and angles of the starting points. Adjusting these factors with the Turing rule set resulted in the three general fingerprint patterns—arches, loops, and whorls—through the varying factors and opposing molecules.
The main goal of this research, however, is to understand how skin matures. This could aid in developing therapies for congenital conditions relating to the skin, allowing for medical regeneration in the future through the artificial reprogramming of skin formation. Such therapies would be most applicable in treating babies with improper skin development such as adermatoglyphia, the absence of fingerprint and toe pad ridges. Such diseases pose problems like delaying the affected individuals from international travel because they cannot be identified without their fingerprints. As a result, the goal for future studies is to understand these skin diseases better and how the molecules involved in skin formation are affected so that they can manipulate these molecules in the future for proper skin development.
On a broader scale, this study doesn’t just show how fingerprints are formed; it helps scientists obtain a comprehensive understanding of how the patterns and systems following Turing’s rules vary between organs and structures. For instance, we can compare the formation of the ridges on mice toes and the ridges on the roofs of mice mouths through the Turing system to understand what molecules distinguish between them. We can observe that though both create ridges, different molecules are used for this process in the two structures. The ridges in the mice’s mouths use FGF as an activator and Shh as an inhibitor, whereas the ridges on the toes use EDAR and WNT as the activators and BMP as the inhibitors. Ultimately, studying these differences to understand how they lead to similar end goals could help further manipulate certain factors to acquire desired results in skin development.
For generations, fingerprints have aided in identifying individuals, mystifying scientists with their elusive development. For the first time, we are beginning to understand the unfamiliar ways in which fingerprints form through Turing’s rules, despite the multifarious other questions that remain. What was their role throughout human evolution? How can we create therapies for those with congenital conditions that prevent them from having properly developed fingerprints and skin structures? Perhaps in the distant future, we’ll have the answers to these questions, as well as technology that can help us reprogram the process of how skin develops.