Shifting the Fight Against Superbugs
Newly-created shapeshifting vancomycin dimers could be the first step in eliminating the threat of superbugs forever.
Reading Time: 4 minutes
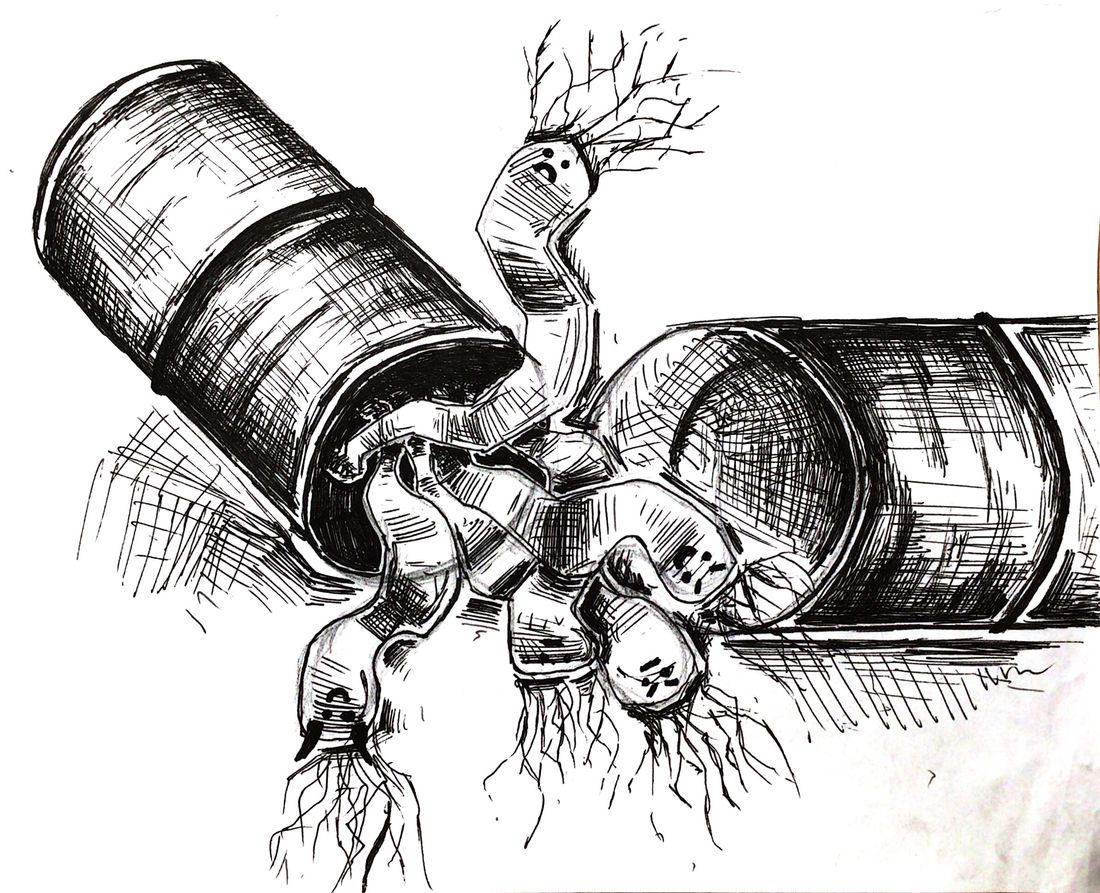
Thanks to monumental innovations in medicine, diseases can now be treated with simple antibiotics. While a case of strep throat might have killed a child in the 19th century, a week of penicillin pills can now cure it. However, as the usage of easily accessible antibiotics becomes more prevalent, the looming threat of antibiotic-resistant bacteria simultaneously becomes more apparent. Our world is facing a new global health crisis, yet there is hope. The rise of new innovations that inhibit superbugs at their source may prove to be the solution to bacterial resistance.
Superbugs, an antibiotic strain of bacteria, have been a global health risk for many years. The World Health Organization declared that antimicrobials are one of the top 10 public health threats to humanity, with the Center for Disease Control estimating that in the U.S. alone, almost three million people are infected and over 35,000 people die from them annually. Impervious to common methods of treatment, these superbugs require new medications. However, pharmaceutical drugs take a long time to develop and can be rendered useless by a single gene mutation. Eliminating superbugs necessitates a permanent solution to an ever-changing problem—a seemingly impossible demand. Yet the key to stopping superbugs could be the very thing that makes them so difficult to overcome: their transformations.
Antibiotics are the cornerstone of modern medicine and are used on a global scale to treat countless diseases. However, it is the widespread use of antibiotics that has become their downfall. Though antibiotics kill most harmful bacteria, they also allow the few genetically resistant strains to survive and proliferate. Over time, this issue compounds with the presence of more resistant strains making antibiotics less effective. The more frequently antibiotics are casually used to treat minor infections, the more prevalent superbugs will become. For example, MRSA, a superbug resistant to beta-lactam antibiotics including all penicillins, is particularly dangerous because it is common in hospitals. In the environment where the most antibiotics are used, superbugs can easily spread and infect immunocompromised patients. For infections that are untreatable by other antibiotics, doctors usually turn to glycopeptides—antibiotics that inhibit cell wall synthesis—specifically, vancomycin.
Vancomycin is an important antibiotic used to treat serious “gram-positive” bacterial infections, or bacteria with thick cell walls, such as Staphylococcus aureus and other bacteria affecting the gastrointestinal tract. It is also a last-resort drug for killing superbugs such as methicillin-resistant Staphylococcus aureus. In the past few decades, however, numerous superbug strains have emerged from this antibiotic, including vancomycin-resistant Staphylococcus aureus and vancomycin-resistant Enterococci (VRE). Vancomycin usually kills bacteria by binding to specific amino acids (D-alanyl-D-alanine moieties) at the ends of polypeptide chains in the peptidoglycan backbone—the substance that makes up the bacterial wall. The enzyme responsible for cross-linking these backbones to form a cohesive unit is then blocked from binding to these terminal ends, inhibiting the formation of the cell wall. VRE develops resistance to vancomycin by changing the critical amino acids in the peptidoglycan backbones, thus blocking the vancomycin molecule from binding to the chain. With this, the enzyme is able to bind and cross-link the backbones, forming the bacterial wall and rendering the antibiotic ineffective. Though a new type of molecule could be made that targets these specific amino acid sequences, it would be a very temporary fix because each change in even one amino acid would demand another new molecule. Instead, a study led by professor and cancer researcher Dr. John Moses found that vancomycin could regain its potency by broadening its capabilities.
Dr. Moses proposed a solution that enables the vancomycin molecule to bind with any needed sequence of amino acids by giving it the ability to change its shape. In order to do this, Dr. Moses joined two vancomycin molecules to a bullvalene “core.” Bullvalene is a fluxional molecule, meaning its chemical bonds can constantly rearrange, in this case, into over 1.2 million different configurations. These newly formed molecules are called shapeshifting vancomycin dimers (SVDs). Since they are not confined to just one shape, SVDs are able to bind more freely to multiple different sequences of amino acids in the peptidoglycan backbone. This leads to improved efficacy against bacteria such as VRE since it is no longer restricted to a single sequence. This not only allows SVDs to kill existing superbugs, but also makes it less likely for other strains of vancomycin-resistant superbugs to emerge from this treatment.
Dr. Moses’s team then conducted tests to assess the effectiveness and virulence of the SVDs and found promising results. This experiment followed the common practice in antibiotic testing of using greater wax moth (Galleria mellonella) larvae as a stand-in for mice or rats due to their similarity to humans in their biological responses to drugs. The larvae were exposed to VRE and split into three experimental groups with differing levels of treatment: no treatment, regular vancomycin treatment, and the SVD treatment. After one week, the survival rate was 10 percent in larvae with no treatment and 40 percent for those treated with regular vancomycin. On the other hand, those undergoing SVD treatment had a survival rate of 70 percent, indicating 75 percent higher success rates than regular vancomycin rates. This implies that SVDs are much more effective at inhibiting the growth of VRE and are more capable of controlling a superbug infection.
The successful creation of SVDs comes with many new possibilities for antibiotics and beyond. Effective treatments for superbugs could save hundreds of thousands of lives per year and remove the need for frequent new therapies. Methods like SVDs could help create a universal flu vaccine and further other incredible developments in medicine. Though more testing is needed to confirm the effectiveness and safety of SVDs, they provide hope for the eventual eradication of superbugs altogether.